The NASA Mars 2020 Mission was launched with the goal of seeking signs of ancient life and collecting samples of rock and regolith (broken rock and soil) for possible return to Earth. Perseverance, the mission’s rover, is testing technologies to help pave the way for future human exploration of Mars. While Perseverance was launched in the summer of 2020, landing on the martian surface on February 18, 2021, the journey started years earlier when the mission’s objectives were outlined, including realistic surface operations, a proof-of-concept instrument suite, and suggestions for threshold science measurements that would meet the proposed objectives. The success of this, as well as past and future missions, is the collective result of thousands of NASA funded projects from teams of researchers and scientists from all over the country that span many decades. University of Michigan Professor Jesse Capecelatro (Mechanical Engineering & Aerospace Engineering) is the lead of one of these projects. In 2016, his research group started working on a project aimed to develop high fidelity models of plume-Induced soil erosion during lunar and planetary landings that will be used in future missions.
During descent, exhaust plumes fluidize surface soil and dust, forming craters and buffeting the lander with coarse, abrasive particles. The SkyCrane technology, used by the Curiosity Rover in 2012 and by Perseverance in 2021, was designed to avoid plume-surface interactions by keeping the jets far above the surface. Regardless of this feature, a wind sensor on NASA’s Mars Curiosity rover was damaged during landing. In the Perseverance’s video footage of the landing, significant erosion and high-speed ejecta were observed. It is also not a practical option for future crewed and sample return missions.
NASA is aiming to improve rovers’ landing gears for future missions. Computational models and simulations are a critical component to achieve this as it is not feasible to mimic the martian or other celestial bodies entry conditions and run thousands of tests in a lab anywhere on Earth. This is where Prof. Capecelatro’s research group, including doctoral candidates Greg Shallcross, and Meet Patel, and postdoctoral fellow Medhi Khalloufi, work comes in as the accurate prediction of surface-plume interactions is necessary for the overall success of future space missions. While simulations of surface-plume interactions have been conducted in the past, these are outdated, and typically relied on simplified assumptions that prevent a detailed and dynamic analysis of the fluid-particle coupling. Capecelatro’s research code will provide NASA with a framework to better predict how different rover designs would impact the landing, specifically the effects of the force of the collision on the planet’s surface, and ability to adjust the rover’s landing trajectory independent of the NASA mission control team on earth.
Prof. Capecelatro’s research project utilizes predictive simulation tools to capture the complex multiphase dynamics associated with rocket exhaust impingement during touchdown. Even in the most powerful supercomputers, a direct solution approach is only capable of accounting for about a thousand particles at the same time, so accurate and predictive multi-scale models of the unresolved flow physics are essential.
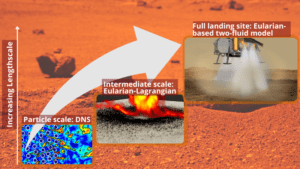
Full landing site image credit: NASA/JPL-Caltech (mars.nasa.gov/resources/24762/mars-sample-return-lander-touchdown-artists-concept/); particle and intermediate scale images: Capecelatro’s Research Group
Particle Scale
The group has been developing the simulation capabilities to directly resolve the flow at the sub-particle scale to shed light on important physics under the extreme conditions relevant to particle-structure interactions. Their model uses a massively parallel compressible particle-laden flow simulation tool where the exhaust plume and its corresponding flow features are computed in an Eulerian-Lagrangian framework. At this scale, for example, the flow between individual particles are resolved, providing important insight on drag and turbulence under these extreme conditions.
Intermediate Scale
As a next step, the particle-scale results inform models used in the intermediate-scale simulations developed by the group, where particles are still tracked individually but the flow is not resolved at a sub-particle resolution, allowing them to simulate upwards of 1 billion particles. At this scale, an Eularian-Lagrangian framework is used to incorporate the ground’s particle flow with the jet’s plume.
Full Landing Site Scale
While the intermediate-scale simulations allow to study erosion and cratering, a full landing site that contains trillions of particles is still out of reach even in the most powerful HPC clusters. After further modeling, Capecelatro’s multi-scale framework will be handed over to NASA where it will be incorporated in simulations of the full landing site. At this scale, NASA’s framework uses an Eularian-based, two fluid model that treats both fluid and particles as a continuum, informed by the particle- and middle-scales models.
Mission Mars 2020 is expanding NASA’s robotic presence on the red planet. While it is a big step to set the stage for future human exploration, the Perseverance Rover needs further redesign to make the voyage safe for humans. Capecelatro’s physics-based models are aiding this task by helping predict and model more accurately the outcomes of a spacecraft attempting to safely land millions of miles from home. As in many other fields, computational science will continue to play a critical role in the future of humanity’s quest to conquer space. #computationalscience everywhere!
Related links:
Sticking the landing on Mars: High-powered computing aims to reduce guesswork
Capecelatro’s Research Group
NASA 2020 Mars Mission: Perseverance Rover